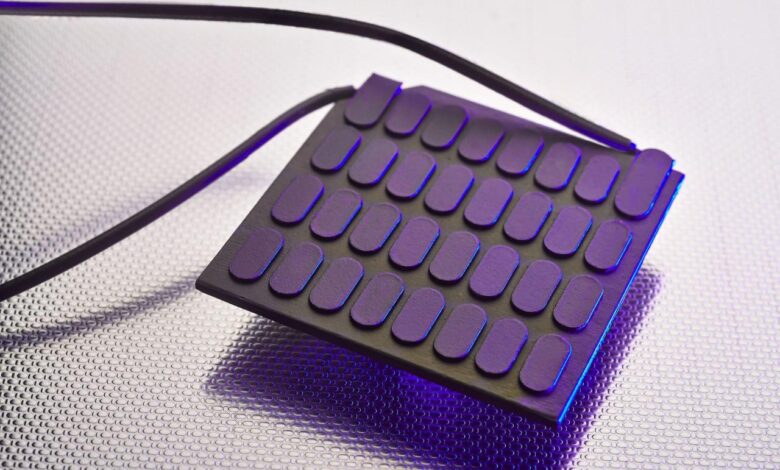
Cheap Materials: Converting Heat to Electricity
Cheap material converts heat to electricity – Cheap materials converting heat to electricity might sound like something out of a science fiction movie, but it’s a very real and promising area of research. Imagine a world where we could harness the heat from our homes, cars, and factories to generate clean energy.
That’s the potential of thermoelectric materials, and it’s a field that’s rapidly evolving.
Thermoelectric materials have the unique ability to convert heat directly into electricity, and vice versa. This process, known as the Seebeck effect, relies on the movement of electrons within the material, driven by temperature differences. While traditional thermoelectric materials have been expensive and inefficient, recent advancements in materials science have led to the development of cheaper and more efficient alternatives, opening up a whole new world of possibilities.
Types of Cheap Thermoelectric Materials
Thermoelectric materials, capable of converting heat energy directly into electricity and vice versa, hold immense potential for a sustainable future. While their applications are numerous, cost-effectiveness is crucial for widespread adoption. This article explores various types of thermoelectric materials, highlighting their advantages, limitations, and cost implications.
Common Thermoelectric Materials, Cheap material converts heat to electricity
Common thermoelectric materials are widely studied and employed in various applications.
Imagine a world where the heat from your car engine could be used to power your phone! Scientists are developing cheap materials that convert heat into electricity, opening up possibilities for renewable energy. But while we wait for that future, let’s see who won and who lost in Tuesday’s elections , which could have a big impact on how we approach clean energy initiatives.
This technology, however, could be a game-changer in the long run, making us less reliant on fossil fuels and paving the way for a more sustainable future.
- Bismuth Telluride (Bi2Te 3) : This material boasts a high thermoelectric figure of merit (ZT) at room temperature, making it suitable for applications like Peltier coolers and power generators. However, its cost and scarcity limit its large-scale use.
- Lead Telluride (PbTe): This material is known for its high ZT at elevated temperatures, making it suitable for waste heat recovery applications. However, its toxicity and cost are concerns.
- Silicon Germanium (SiGe): This material is a popular choice for high-temperature applications, such as radioisotope thermoelectric generators (RTGs) used in spacecraft. It exhibits a high ZT at temperatures above 500°C but is relatively expensive.
Properties, Advantages, and Limitations of Common Materials
The properties, advantages, and limitations of common thermoelectric materials are crucial for determining their suitability for specific applications.
- Bismuth Telluride (Bi2Te 3) : This material offers a high ZT at room temperature, making it suitable for applications like Peltier coolers and power generators. However, its cost and scarcity limit its large-scale use.
- Lead Telluride (PbTe): This material is known for its high ZT at elevated temperatures, making it suitable for waste heat recovery applications. However, its toxicity and cost are concerns.
- Silicon Germanium (SiGe): This material is a popular choice for high-temperature applications, such as radioisotope thermoelectric generators (RTGs) used in spacecraft. It exhibits a high ZT at temperatures above 500°C but is relatively expensive.
Emerging Thermoelectric Materials
The quest for cheaper and more efficient thermoelectric materials has led to research into emerging materials.
- Organic Polymers: These materials offer advantages such as low cost, flexibility, and ease of processing. While their ZT is currently lower than that of traditional materials, significant advancements are being made. For example, recent research has shown that organic polymers can be used to create thermoelectric devices that are flexible and transparent, potentially enabling new applications in wearable electronics and solar energy harvesting.
- Nanomaterials: Nanomaterials exhibit unique properties due to their small size, which can enhance their thermoelectric performance. For example, nanostructured materials like quantum dots and nanowires have shown promising results in improving ZT values. However, the cost and scalability of nanomaterial synthesis remain challenges.
Challenges and Opportunities
The development of cheap thermoelectric materials is a promising area of research with the potential to revolutionize energy harvesting and waste heat recovery. However, several challenges hinder the widespread adoption of these materials, including low efficiency and material stability.Despite these challenges, ongoing research efforts are exploring innovative approaches to enhance the efficiency and cost-effectiveness of thermoelectric materials.
It’s amazing how scientists are finding ways to harness energy from even the most unexpected sources. Like turning heat into electricity with cheap materials! It reminds me of how I’ve switched to reusable cotton produce bags for my grocery shopping, which saves me money and helps the environment.
Just like those reusable bags, these new energy technologies could be a game-changer for sustainability.
These efforts focus on improving material properties, optimizing device design, and developing advanced fabrication techniques.
Efficiency Enhancement
Improving the efficiency of thermoelectric materials is a crucial challenge. The efficiency of a thermoelectric material is determined by its figure of merit (ZT), which is a dimensionless quantity that combines the material’s electrical conductivity, thermal conductivity, and Seebeck coefficient.
A higher ZT value indicates a more efficient thermoelectric material.Current research aims to enhance the ZT of thermoelectric materials through various strategies, including:
- Nanostructuring:Introducing nanostructures into thermoelectric materials can significantly enhance their thermoelectric performance. Nanostructuring can effectively reduce thermal conductivity by scattering phonons, which are the carriers of heat. For example, researchers have successfully demonstrated that incorporating nanoparticles or quantum dots into thermoelectric materials can improve their ZT values.
Imagine a world where the heat from your coffee cup could power your phone! That’s the kind of future that’s possible with the development of cheap materials that convert heat into electricity. While we’re waiting for that breakthrough, we can still indulge in the exciting world of cocktails.
Tales of the Cocktail has just announced the top four finalists for their 16th annual Spirited Awards, celebrating the best in the beverage industry. It’s a reminder that even as we strive for technological advancements, the simple pleasures of a well-crafted drink remain timeless.
Perhaps one day, those drinks will be powered by the heat of our own bodies!
- Alloying and Doping:Modifying the electronic band structure of thermoelectric materials through alloying or doping can enhance their electrical conductivity and Seebeck coefficient. This strategy involves introducing specific elements or impurities into the material’s crystal lattice, altering its electronic properties. For instance, doping bismuth telluride with selenium or antimony can improve its thermoelectric efficiency.
- Multi-Material Systems:Utilizing multi-material systems, such as superlattices or heterostructures, can create materials with improved thermoelectric properties. These systems combine different materials with complementary properties, leading to synergistic effects that enhance thermoelectric performance. For example, researchers have developed superlattices of lead telluride and lead selenide, achieving significantly higher ZT values compared to individual materials.
Material Stability
Another significant challenge is the long-term stability of thermoelectric materials under operating conditions. Thermoelectric devices are often exposed to harsh environments, including high temperatures, corrosive gases, and mechanical stress. These conditions can degrade the material’s performance over time.Research efforts are focused on developing materials with improved stability, such as:
- High-Temperature Stability:Developing materials that can withstand high temperatures without degradation is crucial for applications such as waste heat recovery. Researchers are exploring materials with high melting points and resistance to oxidation. For instance, skutterudite compounds, such as cobalt-based skutterudites, exhibit excellent high-temperature stability and have shown promising thermoelectric performance.
- Chemical Resistance:Thermoelectric materials need to be resistant to corrosive gases and liquids, especially in environments with high humidity or exposure to aggressive chemicals. Researchers are investigating materials with protective coatings or inherently resistant compositions to ensure long-term performance. For example, using ceramic coatings or encapsulating thermoelectric materials within inert matrices can enhance their chemical resistance.
- Mechanical Durability:Thermoelectric materials should be mechanically durable to withstand vibrations, shocks, and other mechanical stresses. Researchers are exploring materials with high fracture toughness and tensile strength, along with innovative fabrication techniques to improve their mechanical properties. For example, using composites or reinforcing thermoelectric materials with fibers can enhance their mechanical durability.
Cost Reduction
The cost of thermoelectric materials is a major barrier to their widespread adoption. The synthesis and fabrication of high-performance thermoelectric materials can be expensive, limiting their use in cost-sensitive applications.Research is actively seeking cost-effective solutions, such as:
- Low-Cost Materials:Exploring alternative materials with comparable thermoelectric properties but lower production costs is a key strategy. Researchers are investigating abundant and inexpensive materials, such as oxides, sulfides, and silicides, which can be synthesized using cost-effective techniques. For instance, copper sulfide has shown promising thermoelectric properties and is a readily available and low-cost material.
- Simplified Fabrication:Developing simplified fabrication techniques that reduce production costs is crucial. Researchers are exploring cost-effective synthesis methods, such as powder metallurgy, spray pyrolysis, and sol-gel processing. These techniques can significantly reduce the overall cost of producing thermoelectric materials.
- Optimized Device Design:Optimizing the design of thermoelectric devices can minimize material usage and enhance their efficiency, leading to cost savings. Researchers are exploring innovative device architectures and geometries that maximize thermoelectric performance while minimizing material consumption. For example, using thin-film technologies or micro-scale devices can reduce material usage and improve overall cost-effectiveness.
Future Prospects: Cheap Material Converts Heat To Electricity
The field of thermoelectric materials is brimming with potential, promising a future where waste heat is transformed into valuable electricity. Research and development efforts are actively exploring new materials, optimizing existing ones, and devising innovative applications to unlock the full potential of this technology.
Impact on Renewable Energy and Sustainable Technology
Thermoelectric materials can play a pivotal role in the transition to a sustainable future by contributing to the development of renewable energy sources and reducing our reliance on fossil fuels.
- Waste Heat Recovery:Thermoelectric generators can capture and convert waste heat from industrial processes, power plants, and even vehicles into electricity, enhancing energy efficiency and reducing carbon emissions. For instance, a thermoelectric generator placed in the exhaust system of a car could generate electricity, powering onboard systems or even feeding back into the vehicle’s battery.
- Hybrid and Electric Vehicles:Thermoelectric materials can be integrated into hybrid and electric vehicles to improve energy efficiency. By capturing waste heat from the engine or brakes, thermoelectric generators can supplement the vehicle’s battery, extending its range and reducing reliance on external charging.
- Renewable Energy Integration:Thermoelectric generators can be incorporated into solar and wind energy systems to improve efficiency and provide a more consistent power supply. For example, a thermoelectric generator could capture waste heat from solar panels, generating additional electricity even when the sun is not shining directly on the panels.
Potential Applications in Everyday Life
Thermoelectric materials have the potential to revolutionize everyday life by enabling new and innovative applications that enhance energy efficiency, comfort, and sustainability.
- Wearable Electronics:Thermoelectric materials can be incorporated into wearable devices to power sensors, displays, and other components using body heat. This could lead to the development of self-powered fitness trackers, smartwatches, and even medical devices.
- Smart Homes:Thermoelectric generators can be integrated into smart home appliances, such as refrigerators, air conditioners, and washing machines, to improve energy efficiency and reduce operating costs. For example, a thermoelectric generator could capture waste heat from a refrigerator’s compressor, generating electricity to power the refrigerator’s internal components.
- Personal Cooling and Heating:Thermoelectric materials can be used to create portable cooling and heating devices that are powered by body heat or environmental temperature differences. This could lead to the development of more efficient and environmentally friendly air conditioners, fans, and heating systems.
Applications Across Different Sectors
The versatility of thermoelectric materials opens doors to diverse applications across various sectors, contributing to a more sustainable and efficient future.
Sector | Potential Applications |
---|---|
Energy | Waste heat recovery, hybrid and electric vehicle power systems, renewable energy integration, thermoelectric generators for power plants and industrial processes |
Electronics | Wearable electronics, smart home appliances, sensors, power management systems, thermoelectric coolers for electronics |
Transportation | Hybrid and electric vehicles, aircraft, trains, ships, thermoelectric generators for waste heat recovery in engines and brakes |
Healthcare | Medical devices, drug delivery systems, biocompatible implants, temperature control systems |
Agriculture | Precision agriculture, greenhouse heating and cooling, irrigation systems, food preservation |
Environmental | Waste heat recovery, air and water purification, environmental monitoring, pollution control |
Epilogue
The development of cheap and efficient thermoelectric materials holds immense potential for a more sustainable future. From recovering waste heat to powering wearable electronics, these materials have the power to revolutionize how we generate and consume energy. As research continues to push the boundaries of what’s possible, we can expect to see even more innovative applications emerge, transforming the way we live and interact with the world around us.