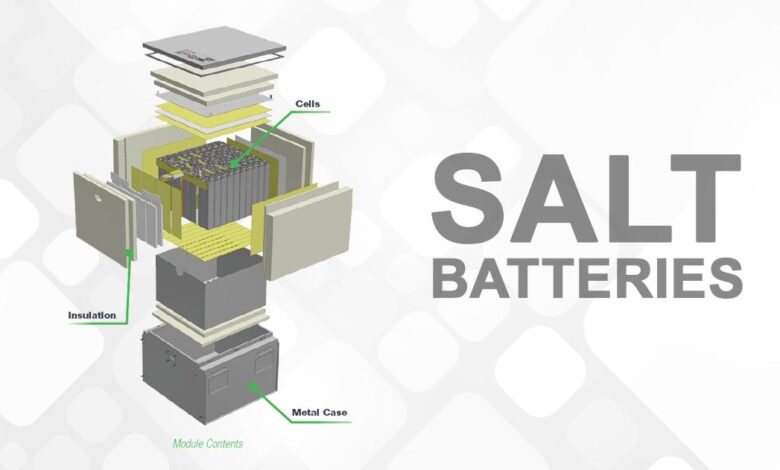
Rechargeable Molten Salt Battery: Freezing Energy for Long-Term Storage
Rechargeable molten salt battery freezes energy in place for long term storage – Rechargeable molten salt battery freezes energy in place for long-term storage, setting the stage for a revolution in energy storage. Imagine a battery that can store energy for months or even years, providing a reliable and sustainable power source for homes, businesses, and entire grids.
Molten salt batteries are not just a futuristic concept; they are a reality with the potential to reshape our energy landscape.
These batteries work by harnessing the power of molten salts, which are heated to high temperatures, allowing them to store and release energy efficiently. This unique approach offers significant advantages over traditional lithium-ion batteries, including longer storage duration, higher energy density, and greater safety.
The ability to store energy for extended periods makes molten salt batteries ideal for applications like grid-scale energy storage, renewable energy integration, and electric vehicle charging.
Introduction to Rechargeable Molten Salt Batteries
Rechargeable molten salt batteries are a promising technology for large-scale energy storage, offering advantages in terms of cost, safety, and lifespan compared to conventional lithium-ion batteries. These batteries utilize molten salts as electrolytes, enabling operation at high temperatures, which enhances their performance and durability.Molten salt batteries operate based on the movement of ions within a molten salt electrolyte.
When charging, an external power source drives ions from one electrode to the other, storing energy in the form of chemical bonds. During discharge, these stored chemical bonds are broken, releasing energy and generating an electric current.
History of Molten Salt Battery Technology
The development of molten salt battery technology can be traced back to the 1960s, with early research focusing on high-temperature applications in aerospace and nuclear power. The first practical molten salt battery was developed in the 1970s by researchers at the Argonne National Laboratory.
These early batteries used a lithium-chloride-potassium-chloride electrolyte and a sulfur-based cathode, achieving high energy densities but with limited cycle life.Subsequent research efforts focused on improving the performance and longevity of molten salt batteries. The use of different electrode materials, electrolyte compositions, and cell designs has led to significant advancements in their energy storage capacity, power output, and cycle life.
Today, molten salt batteries are being explored for various applications, including grid-scale energy storage, electric vehicles, and industrial processes.
Imagine a battery that stores energy like a frozen river, ready to be thawed and released when needed. That’s the promise of rechargeable molten salt batteries, a technology with the potential to revolutionize long-term energy storage. But just like navigating the complexities of job sharing, as highlighted in this Weareteachers post , finding the right balance and overcoming hurdles is crucial for success.
Molten salt batteries, with their ability to store energy for extended periods, offer a solution for bridging the gap between renewable energy sources and consistent power supply, just like finding a harmonious and fulfilling work arrangement is key to a teacher’s well-being.
Key Features and Advantages of Molten Salt Batteries: Rechargeable Molten Salt Battery Freezes Energy In Place For Long Term Storage
Molten salt batteries stand out as a promising energy storage technology due to their unique characteristics and compelling advantages over traditional lithium-ion batteries. These batteries leverage the high energy density and long storage duration of molten salts to offer a compelling solution for addressing the challenges of renewable energy integration and grid stability.
High Energy Density and Long Storage Duration
Molten salt batteries possess an inherent advantage in energy density due to the high ionic conductivity of molten salts. The molten electrolyte allows for the efficient movement of ions, enabling higher charge and discharge rates compared to traditional lithium-ion batteries.
Moreover, the high thermal stability of molten salts allows for long-term storage without significant degradation. The energy stored in a molten salt battery can be retained for extended periods, making it suitable for applications requiring long-duration energy storage.
Advantages over Lithium-Ion Batteries
- Higher Energy Density:Molten salt batteries can store significantly more energy per unit volume compared to lithium-ion batteries. This advantage translates to smaller and more compact battery systems for the same energy capacity.
- Longer Cycle Life:Molten salt batteries exhibit a longer cycle life than lithium-ion batteries, meaning they can withstand a greater number of charge and discharge cycles before experiencing significant performance degradation. This longevity makes them ideal for applications requiring frequent cycling, such as grid-scale energy storage.
- Improved Safety:Molten salt batteries are generally considered safer than lithium-ion batteries. The high melting point of the molten salts reduces the risk of thermal runaway, a common concern with lithium-ion batteries. Moreover, the use of non-flammable electrolytes further enhances safety.
- Lower Cost:The materials used in molten salt batteries are generally less expensive than those used in lithium-ion batteries. This cost advantage can make molten salt batteries a more attractive option for large-scale energy storage applications.
Role in Renewable Energy Integration and Grid Stability
Molten salt batteries play a crucial role in addressing the challenges associated with integrating renewable energy sources into the grid. The intermittent nature of renewable energy sources like solar and wind requires reliable and efficient energy storage solutions. Molten salt batteries, with their high energy density and long storage duration, provide a means to store excess energy generated during peak production periods and release it when demand exceeds supply.
This helps to stabilize the grid, ensuring a consistent and reliable power supply.
Molten Salt Battery Materials and Components
Molten salt batteries are unique in their reliance on high-temperature operation, which necessitates the use of specialized materials that can withstand these extreme conditions. The selection of these materials is crucial for the battery’s performance, longevity, and overall safety.
The core components of a molten salt battery include the electrolyte, electrodes, and the container that houses the entire system. Each of these components plays a critical role in the battery’s functionality, and their specific properties determine the battery’s overall characteristics.
Electrolytes
Electrolytes are the heart of any battery, acting as the medium for ion transport between the electrodes. In molten salt batteries, the electrolyte is a molten salt mixture that remains in a liquid state at elevated temperatures.
The choice of electrolyte is critical, as it directly influences the battery’s operating temperature, electrochemical window, and ionic conductivity. Here are some important properties to consider:
- High ionic conductivity:The electrolyte must efficiently conduct ions to enable rapid charge and discharge cycles. Ionic conductivity increases with temperature, making molten salt electrolytes advantageous in this regard.
- Wide electrochemical window:A wide electrochemical window allows the electrolyte to accommodate a wider range of electrode materials, expanding the potential for higher voltage and energy density.
- Thermal stability:The electrolyte must remain stable at high temperatures to prevent decomposition or reactions that could compromise the battery’s performance or safety.
- Chemical stability:The electrolyte should be chemically inert towards the electrode materials to prevent unwanted reactions that could degrade the battery.
Common molten salt electrolytes include:
- Lithium chloride (LiCl):A commonly used salt in molten salt batteries, offering good ionic conductivity and a wide electrochemical window.
- Lithium fluoride (LiF):Provides high thermal stability and a wide electrochemical window, often used in combination with other salts.
- Sodium chloride (NaCl):A less expensive alternative to lithium salts, but with lower ionic conductivity and a narrower electrochemical window.
Electrodes
Electrodes are the sites where electrochemical reactions occur, converting chemical energy into electrical energy and vice versa. Molten salt batteries typically employ metal-based electrodes, which offer high conductivity and stability at high temperatures.
The key properties of electrode materials are:
- High electrical conductivity:To minimize energy losses during charge and discharge, electrodes need to be highly conductive.
- Electrochemical stability:Electrodes should resist corrosion and degradation in the harsh molten salt environment to maintain their functionality over time.
- Mechanical strength:Electrodes must be mechanically robust to withstand the thermal stresses associated with high-temperature operation.
Common electrode materials include:
- Lithium metal:Offers high theoretical capacity and low density, making it a promising anode material. However, lithium metal’s reactivity and dendrite formation pose challenges.
- Graphite:A well-established anode material with good electrical conductivity and stability, though its capacity is lower than lithium metal.
- Transition metal oxides:Offer higher capacity compared to graphite, but their conductivity and stability can be limited.
Containers, Rechargeable molten salt battery freezes energy in place for long term storage
The container plays a crucial role in containing the molten salt electrolyte and electrodes, preventing leaks and ensuring safe operation. The container material must be chemically inert towards the electrolyte and electrodes, while also withstanding the high temperatures and pressures.
Rechargeable molten salt batteries are a game-changer for long-term energy storage, essentially freezing energy in place for when we need it. It’s a technology that could revolutionize how we power our homes and industries, especially as we transition to renewable energy sources.
But the rise of this technology is also highlighting a complex issue: why unions are growing and shrinking at the same time, as explored in this fascinating article why unions are growing and shrinking at the same time.
This dynamic is crucial to understanding the future of labor in the face of rapid technological advancement, which is exactly what we’re seeing with the development of molten salt batteries. The ability to store energy on a massive scale will reshape our energy landscape, and it’s important to consider the impact on workers and the role of unions in this changing world.
Important properties of container materials include:
- High melting point:The container material should have a melting point higher than the operating temperature of the battery to prevent melting or deformation.
- Chemical inertness:The container material must not react with the electrolyte or electrodes to avoid contamination and performance degradation.
- Mechanical strength:The container must be strong enough to withstand the internal pressure and thermal stresses during operation.
Common container materials include:
- Ceramics:Offer high melting points and chemical inertness, but can be brittle and susceptible to thermal shock.
- Metals:Metals like stainless steel or nickel alloys can offer good mechanical strength and resistance to corrosion, but their melting points may be lower than ceramics.
Challenges in Material Selection
Selecting the right materials for molten salt batteries presents several challenges:
- High-temperature compatibility:Finding materials that can withstand the high temperatures without degrading or reacting with the electrolyte is crucial.
- Corrosion resistance:The harsh environment of molten salts can lead to corrosion of electrode and container materials, requiring careful selection of corrosion-resistant materials.
- Cost:Some materials, like lithium metal and certain ceramics, can be expensive, impacting the overall cost of the battery.
- Long-term stability:Ensuring the materials maintain their performance and stability over extended periods of operation is critical for long-term battery reliability.
Operating Principles and Mechanisms
Molten salt batteries, unlike conventional batteries, operate at high temperatures, leveraging the unique properties of molten salts to store and release energy. The electrochemical processes involved in charging and discharging are crucial to understanding how these batteries work.
Electrochemical Processes in Charging and Discharging
The operation of a molten salt battery involves the reversible transfer of ions between electrodes immersed in a molten salt electrolyte. During charging, an external electrical current forces the movement of ions from one electrode to the other, storing energy in the battery.
The discharge process reverses this flow, releasing stored energy as electricity.
The electrochemical reactions occurring at the electrodes during charging and discharging are dependent on the specific battery chemistry.
For example, in a sodium-sulfur battery, the charging process involves the oxidation of sodium at the anode and the reduction of sulfur at the cathode. This results in the formation of sodium sulfide (Na 2S) at the cathode, storing the energy.
During discharge, the reverse reaction occurs, releasing energy as sodium ions move from the anode to the cathode through the electrolyte.
Role of Temperature and Ion Transport
Temperature plays a crucial role in the operation of molten salt batteries. The high operating temperatures (typically between 300°C and 600°C) ensure that the electrolyte remains in a molten state, facilitating ion transport between the electrodes.
The rate of ion transport, or ionic conductivity, increases with temperature, leading to faster charging and discharging rates.
Imagine a battery that can store energy for months, even years, without losing much of its charge. That’s the promise of rechargeable molten salt batteries, which freeze energy in place for long-term storage. This technology is so promising that even premium whiskey producer Uncle Nearest, known for its traditional approach, is investing in innovation.
They recently poured $5 million into Hella Cocktail, a BIPOC-led non-alcoholic company, through their venture arm, demonstrating a commitment to both sustainability and diversity. This kind of forward-thinking investment could help accelerate the development of molten salt batteries and bring us closer to a future where energy storage is no longer a bottleneck.
The movement of ions through the molten salt electrolyte is essential for the energy storage and release process. The ionic conductivity of the electrolyte determines the efficiency and speed of the battery.
Steps in the Operation of a Molten Salt Battery System
The operation of a molten salt battery system involves a series of steps, from charging to discharge:
- Charging:An external electrical current is applied to the battery, forcing ions to move from the anode to the cathode, storing energy in the battery. The specific electrochemical reactions occurring at the electrodes depend on the battery chemistry.
- Storage:The stored energy is held within the battery in the form of chemical compounds formed during the charging process. The high temperature ensures the electrolyte remains molten, preventing any loss of stored energy due to solidification.
- Discharge:When energy is required, the external circuit is closed, allowing ions to flow back from the cathode to the anode, releasing stored energy as electricity. The electrochemical reactions at the electrodes reverse, restoring the original state of the battery.
Challenges and Future Directions
While molten salt batteries hold immense promise for grid-scale energy storage, several challenges need to be addressed before widespread adoption. These challenges primarily revolve around cost, safety, and scalability, and their resolution is crucial for the technology’s successful integration into the energy landscape.
Cost Reduction
Lowering the cost of molten salt batteries is critical for their widespread adoption. Current production costs are significantly higher than conventional lithium-ion batteries, hindering their competitiveness.
- Material Cost Optimization:Developing alternative, less expensive materials for electrodes, electrolytes, and containers can significantly reduce production costs. Research is focused on exploring cheaper and abundant materials, such as low-cost metal oxides and sulfides, to replace expensive materials like lithium and nickel.
- Manufacturing Process Improvement:Optimizing the manufacturing process, including automation and scaling up production, can reduce labor costs and increase efficiency. Developing efficient and cost-effective methods for producing high-quality components is essential.
- Design Innovation:Innovative designs that minimize material usage and simplify the battery structure can contribute to cost reduction. For example, using thin-film technologies or novel electrode architectures can enhance energy density while reducing material requirements.
Safety Enhancement
Ensuring the safe operation of molten salt batteries is paramount due to the high operating temperatures involved.
- Thermal Management:Developing effective thermal management systems to prevent overheating and thermal runaway is crucial. This can involve using advanced insulation materials, efficient heat dissipation mechanisms, and intelligent control systems to regulate battery temperature.
- Electrolyte Stability:Research focuses on developing electrolytes with enhanced thermal stability and electrochemical stability at high temperatures. This involves investigating new salt compositions and additives that improve ionic conductivity while minimizing corrosion and degradation.
- Container Design:Designing robust and leak-proof containers that can withstand high temperatures and pressures is essential. This includes using high-temperature-resistant materials and innovative sealing techniques to prevent electrolyte leakage and potential hazards.
Scalability and Durability
Scaling up molten salt battery production to meet the demands of grid-scale energy storage requires addressing several challenges.
- Large-Scale Production:Developing efficient and scalable manufacturing processes for large-capacity batteries is crucial. This involves optimizing the design and fabrication of large-scale cells and modules, ensuring consistent performance and reliability.
- Long-Term Durability:Molten salt batteries need to demonstrate long-term durability and stability under demanding operating conditions. Research focuses on improving the lifetime of the battery by addressing issues like electrode degradation, electrolyte decomposition, and corrosion.
- Integration with Grid Systems:Developing effective strategies for integrating molten salt batteries into existing grid systems is essential. This involves optimizing the battery’s power and energy output, ensuring seamless communication and control with the grid, and minimizing the impact on grid stability.
Final Summary
Molten salt batteries are a game-changer in the world of energy storage, offering a compelling solution to the challenges of intermittency and grid stability associated with renewable energy sources. With their high energy density, long storage duration, and inherent safety features, these batteries have the potential to transform our energy future.
As research and development continue, we can expect to see even more innovative applications and advancements in molten salt battery technology, paving the way for a more sustainable and reliable energy landscape.