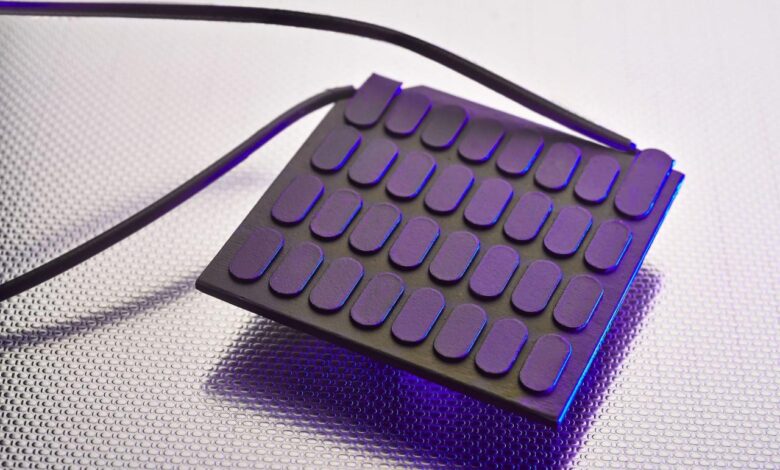
Cheap Material Converts Heat to Electricity: A New Frontier
Cheap material converts heat to electricity, a concept that seems straight out of science fiction, is rapidly becoming a reality. Imagine a world where waste heat from cars, factories, and even our own bodies could be harnessed to power our devices.
This is the promise of thermoelectric materials, and the race is on to find cheap, efficient solutions.
Thermoelectric materials have the incredible ability to directly convert heat into electricity, and vice versa, thanks to the Seebeck and Peltier effects. While traditional thermoelectric materials have been expensive and limited in their efficiency, scientists are now exploring a new generation of materials that are both affordable and effective.
Introduction to Thermoelectric Materials
Thermoelectric materials are fascinating materials that can directly convert heat energy into electrical energy and vice versa. This unique property makes them valuable for a range of applications, from waste heat recovery to solid-state refrigeration.
The Seebeck Effect
The Seebeck effect, discovered in 1821 by Thomas Johann Seebeck, forms the basis of thermoelectric energy generation. When a temperature difference exists across a conductor, a voltage is generated. This voltage is directly proportional to the temperature difference and the material’s Seebeck coefficient.
The Seebeck coefficient (S) is a material property that quantifies the voltage generated per unit temperature difference.
Imagine harnessing the warmth of the sun to power your devices, all thanks to a cheap material that converts heat into electricity. It’s a concept that seems straight out of a sci-fi movie, but researchers are making strides in this area.
And while we wait for that technology to become mainstream, we can still enjoy the sun’s warmth in a luxurious setting like the Ritz-Carlton Lake Tahoe , a summer mountain paradise. After all, a little R&R can be just as energizing as any new invention!
This effect can be visualized as follows:Imagine a conductor with two ends at different temperatures. The hot end experiences increased electron activity, leading to a higher concentration of electrons. This imbalance in electron density creates an electric field that drives electrons towards the colder end.
This flow of electrons constitutes an electric current.
The Peltier Effect
The Peltier effect, discovered in 1834 by Jean-Charles Peltier, is the reverse of the Seebeck effect. When an electric current flows through a junction of two dissimilar conductors, heat is either absorbed or released at the junction. The direction of heat flow depends on the direction of the current and the materials’ Peltier coefficients.
Imagine a world where even the heat from your coffee cup could power your phone! Scientists are developing cheap materials that can convert heat into electricity, a game-changer for renewable energy. This breakthrough could have huge implications for the future, just like the potential consequences of a Pelosi trip to Taiwan, which would test China’s appetite for confrontation.
The development of these new materials could lead to a more sustainable and energy-independent future, while the geopolitical situation in the region remains a delicate balancing act.
The Peltier coefficient (Î ) represents the amount of heat absorbed or released per unit electric current.
For example, if a current flows from a material with a higher Peltier coefficient to a material with a lower Peltier coefficient, heat is absorbed at the junction, resulting in a cooling effect. Conversely, if the current flows in the opposite direction, heat is released, leading to a heating effect.
Examples of Thermoelectric Materials and Their Applications
Thermoelectric materials are classified based on their ability to convert heat energy into electrical energy (n-type) or electrical energy into heat energy (p-type). Some common examples include:
- Bismuth Telluride (Bi2Te 3): This material is widely used in room-temperature thermoelectric devices due to its high Seebeck coefficient and relatively low thermal conductivity. It finds applications in thermoelectric generators for waste heat recovery and thermoelectric coolers for small-scale refrigeration.
- Lead Telluride (PbTe):This material is suitable for higher temperature applications (up to 500°C). It is used in thermoelectric generators for power generation from industrial waste heat and in thermoelectric coolers for high-temperature applications.
- Silicon Germanium (SiGe):This material is used in radioisotope thermoelectric generators (RTGs) for space applications. RTGs utilize the heat generated from radioactive decay to produce electricity, making them ideal for long-duration missions where solar energy is unavailable.
Cheap Materials for Thermoelectric Conversion
Thermoelectric materials convert heat energy directly into electrical energy and vice versa. While some materials like bismuth telluride and lead telluride offer excellent thermoelectric efficiency, they are often expensive and have limited availability. The quest for affordable and abundant materials for thermoelectric applications is ongoing, and significant progress has been made in identifying and developing cheap materials with promising thermoelectric properties.
Commonly Used Cheap Materials, Cheap material converts heat to electricity
These materials offer a cost-effective alternative to traditional thermoelectric materials, while still maintaining reasonable thermoelectric efficiency.
- Silicon Germanium (SiGe):SiGe alloys are widely used in high-temperature applications due to their stability and relatively high thermoelectric efficiency. They are relatively inexpensive and readily available, making them a popular choice for applications like waste heat recovery.
- Lead Telluride (PbTe):PbTe is a well-known thermoelectric material with a high figure of merit (ZT) at moderate temperatures. Although it is a lead-based material, it is still considered a cheaper alternative to some other high-performance materials.
- Skutterudites:Skutterudites are a class of intermetallic compounds with the general formula CoSb 3. They offer high thermoelectric efficiency at moderate temperatures and are known for their good thermal stability. These materials are gaining increasing attention due to their potential for cost-effective applications.
Imagine a world where even the heat from your coffee mug could power your phone! Scientists are working on cheap materials that can convert heat directly into electricity, and it’s pretty exciting. This reminds me of how Chef Nikhil Abuvala believes travel is the best cooking teacher, for chef nikhil abuvala travel is the best cooking teacher , because it exposes you to new ingredients, techniques, and cultures.
Just like travel broadens culinary horizons, this new technology could revolutionize how we harness energy, opening up possibilities we never thought possible.
- Tin Selenide (SnSe):SnSe is a promising thermoelectric material with a high ZT at high temperatures. Its low cost and abundance make it a potential candidate for applications in energy harvesting and waste heat recovery.
- Copper Sulfide (Cu2S): Cu 2S is a cheap and abundant material with a relatively high thermoelectric efficiency at low temperatures. It is being investigated for applications in flexible thermoelectric devices and energy harvesting from body heat.
Comparison of Properties and Thermoelectric Efficiency
The thermoelectric efficiency of a material is primarily determined by its figure of merit (ZT), which is a dimensionless quantity that combines the material’s electrical conductivity, thermal conductivity, and Seebeck coefficient.
- SiGe alloyshave a ZT of around 1 at high temperatures, making them suitable for applications like waste heat recovery in automotive and industrial settings.
- PbTehas a ZT of around 1.5 at moderate temperatures, making it a suitable choice for applications like thermoelectric generators.
- Skutteruditeshave a ZT of around 1.8 at moderate temperatures, making them highly efficient for applications like power generation from waste heat.
- SnSeexhibits a ZT of around 2.5 at high temperatures, making it a promising material for high-temperature applications.
- Cu2S has a ZT of around 0.5 at low temperatures, making it suitable for applications like flexible thermoelectric devices and energy harvesting from body heat.
Challenges and Limitations of Using Cheap Materials
Despite their potential, cheap materials often face challenges in achieving high thermoelectric efficiency and long-term stability.
- Lower Thermoelectric Efficiency:Cheap materials often have lower thermoelectric efficiency compared to traditional materials like bismuth telluride. This is due to their lower Seebeck coefficient and higher thermal conductivity.
- Stability and Durability:Some cheap materials may have limited stability and durability at high temperatures or under harsh environmental conditions. This can limit their application in certain industries.
- Processing and Manufacturing:Processing and manufacturing of cheap materials can be challenging due to their complex chemical compositions and physical properties. This can increase the cost of production and hinder their widespread adoption.
- Performance Degradation:Cheap materials may experience performance degradation over time due to factors like oxidation, corrosion, or phase transformation. This can limit their long-term performance and reliability.
The development of cheap and efficient thermoelectric materials is essential for the widespread adoption of thermoelectric technologies. Researchers are continuously exploring new materials and strategies to improve the thermoelectric performance of cheap materials, overcoming the challenges and limitations associated with their use.
Methods for Enhancing Thermoelectric Performance
The quest for efficient thermoelectric materials is fueled by the desire to harness waste heat and convert it into usable electricity. While cheap materials offer a promising avenue, their intrinsic thermoelectric properties often fall short of ideal performance. To bridge this gap, various methods have been developed to enhance the thermoelectric efficiency of these materials.
Doping
Doping involves introducing impurities into the material’s crystal structure to alter its electronic properties. This technique is widely employed to optimize the Seebeck coefficient, electrical conductivity, and thermal conductivity.
- Seebeck coefficient:Doping can increase the Seebeck coefficient by creating a higher concentration of charge carriers, thereby enhancing the voltage generated across the material due to a temperature difference. For example, doping bismuth telluride (Bi 2Te 3) with selenium (Se) increases the Seebeck coefficient by creating more holes, resulting in a higher thermoelectric power output.
- Electrical conductivity:Doping can also enhance electrical conductivity by increasing the number of charge carriers. This is particularly beneficial for materials with inherently low conductivity, as it improves the flow of electricity through the material. For instance, doping lead telluride (PbTe) with sodium (Na) significantly improves its electrical conductivity, enabling more efficient charge transport and increased power generation.
- Thermal conductivity:While doping can enhance electrical conductivity, it can also increase thermal conductivity, which is detrimental to thermoelectric performance. This is because higher thermal conductivity leads to faster heat dissipation, reducing the temperature difference across the material and limiting the thermoelectric conversion efficiency.
To mitigate this, researchers often employ strategies like alloying or introducing nano-sized precipitates to scatter phonons (heat carriers), reducing thermal conductivity without significantly impacting electrical conductivity.
Nanostructuring
Nanostructuring refers to manipulating the material’s structure at the nanoscale, creating unique properties that enhance thermoelectric performance.
- Seebeck coefficient:Nanostructuring can enhance the Seebeck coefficient by increasing the density of states at the Fermi level, leading to a greater response to temperature gradients. This is often achieved by creating quantum wells or superlattices within the material, effectively manipulating the energy levels of charge carriers and enhancing the Seebeck coefficient.
- Electrical conductivity:Nanostructuring can also improve electrical conductivity by creating pathways for efficient charge transport. For example, introducing nanowires or quantum dots into the material can create interconnected pathways for electrons, reducing resistance and increasing electrical conductivity.
- Thermal conductivity:Nanostructuring can significantly reduce thermal conductivity by creating interfaces and boundaries that scatter phonons, impeding heat flow. This is a key advantage of nanostructured materials, as it allows for higher temperature gradients and improved thermoelectric efficiency. For instance, introducing nanowires or quantum dots into the material can create phonon scattering centers, reducing thermal conductivity without significantly affecting electrical conductivity.
Material Synthesis
The method used to synthesize the material can significantly influence its thermoelectric properties.
- Seebeck coefficient:Controlled synthesis methods can optimize the Seebeck coefficient by ensuring a specific crystal structure, grain size, and defect concentration. For example, using a sol-gel method to synthesize bismuth telluride can control the crystal structure and grain size, leading to a higher Seebeck coefficient and improved thermoelectric performance.
- Electrical conductivity:Synthesis techniques can also impact electrical conductivity by controlling the purity, defect concentration, and phase composition of the material. For instance, using a melt-spinning technique to synthesize lead telluride can produce materials with reduced defect concentration and improved electrical conductivity.
- Thermal conductivity:The synthesis method can also influence thermal conductivity by controlling the grain size, porosity, and defect concentration. For example, using a powder metallurgy method to synthesize bismuth telluride can result in a higher porosity, leading to reduced thermal conductivity and improved thermoelectric performance.
End of Discussion: Cheap Material Converts Heat To Electricity
The potential of cheap thermoelectric materials is vast, with applications ranging from powering wearable electronics to generating clean energy. As research continues to advance, we can expect to see even more innovative uses for these materials, leading to a future where waste heat is transformed into a valuable resource.
The quest for cheaper, more efficient thermoelectric materials is a fascinating journey, one that holds the promise of a cleaner, more sustainable future.